Alpha motor neuron | |
---|---|
![]() Alpha motor neurons are derived from the basal plate (basal lamina) of the developing embryo. | |
Identifiers | |
NeuroLex ID | sao1154704263 |
TH | H2.00.01.0.00008 |
FMA | 83664 |
Anatomical terms of neuroanatomy |
Alpha (α) motor neurons (also called alpha motoneurons), are large, multipolar lower motor neurons of the brainstem and spinal cord. They innervate extrafusal muscle fibers of skeletal muscle and are directly responsible for initiating their contraction. Alpha motor neurons are distinct from gamma motor neurons, which innervate intrafusal muscle fibers of muscle spindles.
While their cell bodies are found in the central nervous system (CNS), α motor neurons are also considered part of the somatic nervous system—a branch of the peripheral nervous system (PNS)—because their axons extend into the periphery to innervate skeletal muscles.
An alpha motor neuron and the muscle fibers it innervates comprise a motor unit. A motor neuron pool contains the cell bodies of all the alpha motor neurons involved in contracting a single muscle.
YouTube Encyclopedic
-
1/5Views:174 77452 6732 398304 78527 816
-
Motor neurons | Muscular-skeletal system physiology | NCLEX-RN | Khan Academy
-
Alpha and Gamma Motorneurons: Understanding the Differences
-
17.2 Alpha Gamma Co Activation
-
Muscle stretch reflex | Organ Systems | MCAT | Khan Academy
-
Nervous system 1, Motor neuron
Transcription
When we're at a dance party, and we've determined that this is an appropriate time to dougie, how does our brain tell our body to do so? Well, in this video, we're going to be talking about motor neurons. Motor neurons. These are the nerve cells that come from our brain, go to our bodies, and tell our muscles that it's time to contract . So let's start from the top. In the brain, we have what's called an upper motor neuron. I'll just write "upper motor neuron" right here. An upper motor neuron sends a signal over to a lower motor neuron, that I'll abbreviate right here-- lower motor neuron. And I promise I'm going to draw this out in greater detail in a moment, but just to kind of get an idea of what they do, the lower motor neuron is the direct messenger to muscle to tell it that it's time to start contracting. The upper motor neuron, because it's sending a signal to the lower motor neuron, has two jobs. One, it'll also have the job to tell muscle eventually that it's time to start contracting. The other job it has is that it has to tell lower motor neurons when it's time to stop. It'll tell them that there's no more signal coming in so you should stop telling muscles to start contracting. So that's why upper motor neurons have two functions. Let's take a look at a lower motor neuron in better detail now. The first thing I like to draw here is called the soma. The soma is just the body of the lower motor neuron. The part of the lower motor neuron that receives the signal from the upper motor neuron is kind of branched like this. And there are multiple projections that come off right here. These guys are called dendrites, and they're going to receive the signal. It's where a signal is going to dock on our motor neuron. And after it docks the dendrite, passes through this station, the soma, the cell body, it's cast away along an axon that I'm drawing right here. And I've drawn this extra long because there's going to be a lot going on here before this slab of meat here, or muscle, receives a message. So this is our muscle, the motor end plate, or the muscle that's going to receive information from our axon right here. So one more time, when we're at a dance party and we decide that it's time to shake a leg, how do we tell our leg it's time to shake? Well, we have this signal that's generated from the brain, travels down the upper motor neuron, and then it goes to this lower motor neuron right here. And the way I like to think about a signal, it's kind of like how I think about ships in the Navy. So if this little ship right here is our signal, and it comes from our upper motor neuron, it needs to have somewhere it can dock on this lower motor neuron. That's what dendrites are for. This is where a signal is going to dock. Once it docks there, it has to pass through some type of station, this naval station, which is what the soma is. And after it passes through the station, it's going to move down the axon where it's cast away. The axon is where a signal is cast away from this motor neuron before it ends up going to muscle. But what could happen here? What problem could probably arise, considering how long this axon actually is? Well, the signal could die off. It may not be able to make it all the way to the end. And that's kind of what would happen if you had a lower motor neuron injury or a lesion. If something were to happen to our lower motor neuron, we wouldn't be able to tell a muscle it's time to start contracting. Instead, you would have weakness because your muscle would not be able to contract. The same thing happens when you have an upper motor neuron injury. You're also going to see weakness because you're not able to tell the lower motor neuron it's time to tell the muscle it's supposed to start contracting. But that's not the key characteristic we see with upper motor neuron injuries. The key thing we look for is that we're not able to tell lower motor neurons to stop what they're doing. And so because of that, the lower motor neuron just keeps on telling this muscle, hey, go ahead and contract. I'm not receiving any signal from here to tell me to stop. And so because of that, this muscle is just going to continuously, spastically contract. And so a key symptom or a key characteristic of an upper motor neuron injury that you don't see with lower motor neuron injuries is spasticity. And so that's what could happen if you have dissipation of a signal at the upper motor neuron. But nature has planned for this. What does she do to make sure that we don't have our signals dissipated? Well, she insulates our neurons. So I'm going to draw three insulating cells right here that wrap the round our axonal fiber right here, or this axon. And these three cells-- I'll label one right here. This is a single cell. It's known as a myelin sheath. That's an I. Myelin sheath. What does that mean? Well, myelin just means "fatty." So, yo mama's so myelin. They mean the same thing. Myelin sheath. And so we cover up the axon fiber to help insulate it, so instead of a signal dying off, it's able to go all the way to the end over here. And depending on where you have this myelin sheath cell in the body, there's a different name. In our central nervous system, which is strictly just the brain and spinal cord, we call them oligodendrocytes. And that's only in the brain and the spinal cord. In the peripheral nervous system, which is literally everything else, any other nerve in our body that's not in the brain or the spinal cord, we call these myelin sheath cells Schwann cells. So I've drawn three up here, and what's going to happen is that you're not going to have dissipation of your signal. No. Instead it's going to continue on to this myelin cell and then jump and land on this node right here, and it's going to jump again. And it keeps doing this from node to node, or this empty space to empty space, until it finally makes it to the end of this axon, or what we call the axon terminal right here. And so this space right here, this open, empty area that has nothing in it, has a specific name. We call this the node of Ranvier, named after probably the smartest scientist ever because he got his name on a scientific structure that literally has nothing. This is just empty space right here. And so as our signal is going to propagate down this axon here and jump from node to node, eventually it'll finally make it to our axon terminal right here, where we can then relay a message to our muscle that it's time to contract. And that's how our motor neurons work.
Location
Alpha motor neurons (α-MNs) innervating the head and neck are found in the brainstem; the remaining α-MNs innervate the rest of the body and are found in the spinal cord. There are more α-MNs in the spinal cord than in the brainstem, as the number of α-MNs is directly proportional to the amount of fine motor control in that muscle. For example, the muscles of a single finger have more α-MNs per fibre, and more α-MNs in total, than the muscles of the quadriceps, which allows for finer control of the force a finger applies.
In general, α-MNs on one side of the brainstem or spinal cord innervate muscles on that same side of the body. An exception is the trochlear nucleus in the brainstem, which innervates the superior oblique muscle of the eye on the opposite side of the face.
Brainstem
In the brainstem, α-MNs and other neurons reside within clusters of cells called nuclei, some of which contain the cell bodies of neurons belonging to the cranial nerves. Not all cranial nerve nuclei contain α-MNs; those that do are motor nuclei, while others are sensory nuclei. Motor nuclei are found throughout the brainstem—medulla, pons, and midbrain—and for developmental reasons are found near the midline of the brainstem.
Generally, motor nuclei found higher in the brainstem (i.e., more rostral) innervate muscles that are higher on the face. For example, the oculomotor nucleus contains α-MNs that innervate muscles of the eye, and is found in the midbrain, the most rostral brainstem component. By contrast, the hypoglossal nucleus, which contains α-MNs that innervate the tongue, is found in the medulla, the most caudal (i.e., towards the bottom) of the brainstem structures.
Spinal cord

In the spinal cord, α-MNs are located within the gray matter that forms the ventral horn. These α-MNs provide the motor component of the spinal nerves that innervate muscles of the body.

As in the brainstem, higher segments of the spinal cord contain α-MNs that innervate muscles higher on the body. For example, the biceps brachii muscle, a muscle of the arm, is innervated by α-MNs in spinal cord segments C5, C6, and C7, which are found rostrally in the spinal cord. On the other hand, the gastrocnemius muscle, one of the muscles of the leg, is innervated by α-MNs within segments S1 and S2, which are found caudally in the spinal cord.
Alpha motor neurons are located in a specific region of the spinal cord's gray matter. This region is designated lamina IX in the Rexed lamina system, which classifies regions of gray matter based on their cytoarchitecture. Lamina IX is located predominantly in the medial aspect of the ventral horn, although there is some contribution to lamina IX from a collection of motor neurons located more laterally. Like other regions of the spinal cord, cells in this lamina are somatotopically organized, meaning that the position of neurons within the spinal cord is associated with what muscles they innervate. In particular, α-MNs in the medial zone of lamina IX tend to innervate proximal muscles of the body, while those in the lateral zone tend to innervate more distal muscles. There is similar somatotopy associated with α-MNs that innervate flexor and extensor muscles: α-MNs that innervate flexors tend to be located in the dorsal portion of lamina IX; those that innervate extensors tend to be located more ventrally.
Development
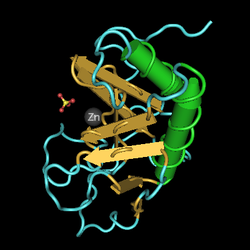
Alpha motor neurons originate in the basal plate, the ventral portion of the neural tube in the developing embryo. Sonic hedgehog (Shh) is secreted by the nearby notochord and other ventral structures (e.g., the floor plate), establishing a gradient of highly concentrated Shh in the basal plate and less concentrated Shh in the alar plate. Under the influence of Shh and other factors, some neurons of the basal plate differentiate into α-MNs.
Like other neurons, α-MNs send axonal projections to reach their target extrafusal muscle fibers via axon guidance, a process regulated in part by neurotrophic factors released by target muscle fibers. Neurotrophic factors also ensure that each muscle fiber is innervated by the appropriate number of α-MNs. As with most types of neurons in the nervous system, α-MNs are more numerous in early development than in adulthood. Muscle fibers secrete a limited amount of neurotrophic factors capable of sustaining only a fraction of the α-MNs that initially project to the muscle fiber. Those α-MNs that do not receive sufficient neurotrophic factors will undergo apoptosis, a form of programmed cell death.
Because they innervate many muscles, some clusters of α-MNs receive high concentrations of neurotrophic factors and survive this stage of neuronal pruning. This is true of the α-MNs innervating the upper and lower limbs: these α-MNs form large cell columns that contribute to the cervical and lumbar enlargements of the spinal cord. In addition to receiving neurotrophic factors from muscles, α-MNs also secrete a number of trophic factors to support the muscle fibers they innervate. Reduced levels of trophic factors contributes to the muscle atrophy that follows an α-MN lesion.
Connectivity
Like other neurons, lower motor neurons have both afferent (incoming) and efferent (outgoing) connections. Alpha motor neurons receive input from a number of sources, including upper motor neurons, sensory neurons, and interneurons. The primary output of α-MNs is to extrafusal muscle fibers. This afferent and efferent connectivity is required to achieve coordinated muscle activity.
Afferent input
UMN origin | α-MN target | Tract name |
---|---|---|
Cerebral cortex | Brainstem | Corticonuclear tract |
Cerebral cortex | Spinal cord | Corticospinal tract |
Red nucleus | Spinal cord | Rubrospinal tract |
Vestibular nuclei | Spinal cord | Vestibulospinal tract |
Midbrain tectum | Spinal cord | Tectospinal tract |
Reticular formation | Spinal cord | Reticulospinal tract |
Upper motor neurons (UMNs) send input to α-MNs via several pathways, including (but not limited to) the corticonuclear, corticospinal, and rubrospinal tracts. The corticonuclear and corticospinal tracts are commonly encountered in studies of upper and lower motor neuron connectivity in the control of voluntary movements.
The corticonuclear tract is so named because it connects the cerebral cortex to cranial nerve nuclei. (The corticonuclear tract is also called the corticobulbar tract, as the target in the brainstem—which is medulla—is archaically called the "bulb.") It is via this pathway that upper motor neurons descend from the cortex and synapse on α-MNs of the brainstem. Similarly, UMNs of the cerebral cortex are in direct control of α-MNs of the spinal cord via the lateral and ventral corticospinal tracts.
The sensory input to α-MNs is extensive and has its origin in Golgi tendon organs, muscle spindles, mechanoreceptors, thermoreceptors, and other sensory neurons in the periphery. These connections provide the structure for the neural circuits that underlie reflexes. There are several types of reflex circuits, the simplest of which consists of a single synapse between a sensory neuron and a α-MNs. The knee-jerk reflex is an example of such a monosynaptic reflex.
The most extensive input to α-MNs is from local interneurons, which are the most numerous type of neuron in the spinal cord. Among their many roles, interneurons synapse on α-MNs to create more complex reflex circuitry. One type of interneuron is the Renshaw cell.
Efferent output
Alpha motor neurons send fibers that mainly synapse on extrafusal muscle fibers. Other fibers from α-MNs synapse on Renshaw cells, i.e. inhibitory interneurons that synapse on the α-MN and limit its activity in order to prevent muscle damage.
Signaling
Like other neurons, α-MNs transmit signals as action potentials, rapid changes in electrical activity that propagate from the cell body to the end of the axon. To increase the speed at which action potentials travel, α-MN axons have large diameters and are heavily myelinated by both oligodendrocytes and Schwann cells. Oligodendrocytes myelinate the part of the α-MN axon that lies in the central nervous system (CNS), while Schwann cells myelinate the part that lies in the peripheral nervous system (PNS). The transition between the CNS and PNS occurs at the level of the pia mater, the innermost and most delicate layer of meningeal tissue surrounding components of the CNS.
The axon of an α-MN connects with its extrafusal muscle fiber via a neuromuscular junction, a specialized type of chemical synapse that differs both in structure and function from the chemical synapses that connect neurons to each other. Both types of synapses rely on neurotransmitters to transduce the electrical signal into a chemical signal and back. One way they differ is that synapses between neurons typically use glutamate or GABA as their neurotransmitters, while the neuromuscular junction uses acetylcholine exclusively. Acetylcholine is sensed by nicotinic acetylcholine receptors on extrafusal muscle fibers, causing their contraction.
Like other motor neurons, α-MNs are named after the properties of their axons. Alpha motor neurons have Aα axons, which are large-caliber, heavily myelinated fibers that conduct action potentials rapidly. By contrast, gamma motor neurons have Aγ axons, which are slender, lightly myelinated fibers that conduct less rapidly.
Clinical significance

Injury to α-MNs is the most common type of lower motor neuron lesion. Damage may be caused by trauma, ischemia, and infection, among others. In addition, certain diseases are associated with the selective loss of α-MNs. For example, poliomyelitis is caused by a virus that specifically targets and kills motor neurons in the ventral horn of the spinal cord. Amyotropic lateral sclerosis likewise is associated with the selective loss of motor neurons.
Paralysis is one of the most pronounced effects of damage to α-MNs. Because α-MNs provide the only innervation to extrafusal muscle fibers, losing α-MNs effectively severs the connection between the brainstem and spinal cord and the muscles they innervate. Without this connection, voluntary and involuntary (reflex) muscle control is impossible. Voluntary muscle control is lost because α-MNs relay voluntary signals from upper motor neurons to muscle fibers. Loss of involuntary control results from interruption of reflex circuits such as the tonic stretch reflex. A consequence of reflex interruption is that muscle tone is reduced, resulting in flaccid paresis. Another consequence is the depression of deep tendon reflexes, causing hyporeflexia.
Muscle weakness and atrophy are inevitable consequences of α-MN lesions as well. Because muscle size and strength are related to the extent of their use, denervated muscles are prone to atrophy. A secondary cause of muscle atrophy is that denervated muscles are no longer supplied with trophic factors from the α-MNs that innervate them. Alpha motor neuron lesions also result in abnormal EMG potentials (e.g., fibrillation potentials) and fasciculations, the latter being spontaneous, involuntary muscle contractions.
Diseases that impair signaling between α-MNs and extrafusal muscle fibers, namely diseases of the neuromuscular junction have similar signs to those that occur with α-MN disease. For example, myasthenia gravis is an autoimmune disease that prevents signaling across the neuromuscular junction, which results in functional denervation of muscle.
See also
References
- John A. Kiernan (2005). Barr's the Human Nervous System: An Anatomical Viewpoint (8th ed.). Hagerstown, MD: Lippincott Williams & Wilkins. ISBN 0-7817-5154-3.
- Duane E. Haines (2004). Neuroanatomy: An Atlas of Structures, Sections, and Systems (6th ed.). Hagerstown, MD: Lippincott Williams & Wilkins. ISBN 0-7817-4677-9.
External links
- NIF Search - Alpha Motor Neuron Archived 2016-03-04 at the Wayback Machine via the Neuroscience Information Framework
